Introduction: the rise of battery storage in power systems
A few years ago, most batteries in our portable applications were still non-rechargeable and played a very minor role in the lives of most people: radio-controlled cars, camping lanterns, kitchen scales… Although rechargeable lead-acid batteries have been around since the mid-19th century, their low energy density (or heavyweight per amount of energy storage) traditionally limited their fields of application. Until 2000, lead-acid batteries dominated the battery market, mostly due to their widespread use as car starter batteries, or their stationary deployment in remote power supply systems (i.e., for remote telecom antennas or off-grid applications). In recent years, electrochemical energy storage technologies have undergone an incredible technical (r)evolution, that allowed a huge increase in power and energy densities, longer lifetime and cyclability, and minimization of safety issues, thus paving the way for several new applications.
Today, it is common for everyone to check, before leaving home every morning, if the mobile phone, the tablet, and/or the laptop is charged… and soon, it will most probably be part of the daily routine to check whether the car is charged, too. Hence, it is clear that batteries have been an essential driver for the Information (IT) revolution and are set to become a key enabler for the paradigm shift within personal transportation towards electric mobility. It is probably for those reasons, that the Nobel Prize in Chemistry was recently (2019) awarded to the researchers that invented the first lithium-ion batteries in the 1990s (J. Goodenough, M.S. Whittingham, A. Yoshino).
Less known by most people, though, is that (large-scale) energy storage systems have started as well to play a very relevant role in our electric power systems in the last decade, and that batteries are a key element to enable the energy transition, essential for the accommodation of high shares of variable renewable energy sources. While batteries and other energy storage technologies still face regulatory and legal challenges to integrate into the traditional power system structure (generation, transport, consumption) and existing markets, a great number of grid-scale battery systems have already been deployed throughout Europe. They contribute, among others, to the stabilization of the grid frequency (at a nominal value of 50Hz) by balancing at all time small gaps between the volume of power generation and the momentaneous power consumption.
What are the reasons for becoming a PV-battery prosumer?
Buildings are central to the EU’s energy policies, as they account for almost 40% of final energy consumption. Hence, the new Energy Performance of Buildings Directive (EPBD) demands the building stock to achieve a “nearly-zero energy balance” (nZEB) condition, mandatory for all new buildings from December 2020 onwards. Rooftop or building-integrated PV is with no doubt the most competitive renewable source for the local generation of electrical power; and batteries are the best option to locally alleviate the issues associated with the mismatch between the solar cycle (with a pronounced midday peak) and the typical energy consumption profile (typically resembling a camel contour, with morning and evening peaks).
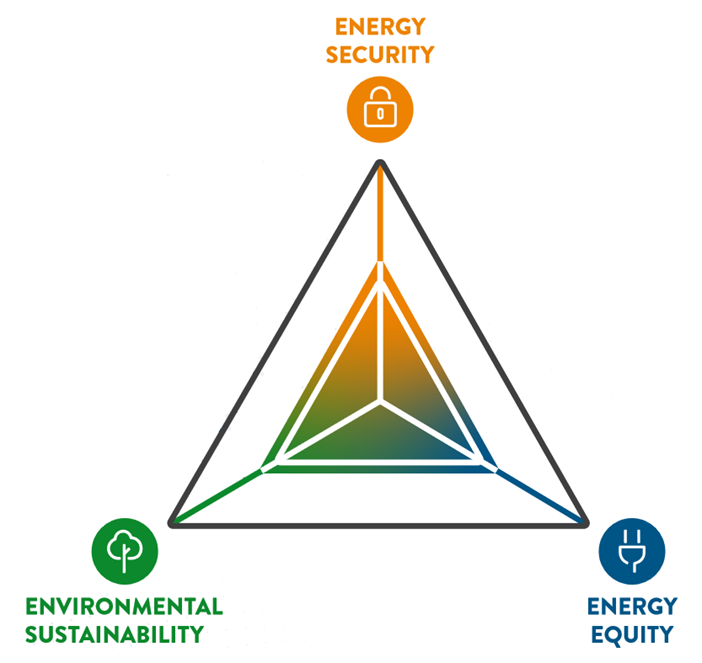
Fig 1: The Energy Trilemma: The three variables that cannot be thought of independently. This index is used by the World Energy Council to evaluate the quality or health of an energy system of a country or region. Picture reprinted and edited from the report “World Energy Trilemma Index, World Energy Council, 2020”
In the following table, we try to briefly address the contribution that the adoption of residential battery storage systems can provide to each of the aspects suggested by the famous Energy Trilemma (see Fig. 1).[1]
Energy Security – Increased power supply security due to enhanced autarky, decreased grid stress For the customer While the self-sufficiency rate (or energy autarky) of households with PV-only is limited to about 30-40%, this can easily increase to about 60-70% with the adoption of a properly sized battery storage. Besides, battery storage systems can eventually provide uninterrupted power supply (UPS) functionalities to maintain power supply in case of grid failures. For the system/society Due to their fast response and high-power capabilities, batteries are one of the best candidates to provide stability to the electrical power system. Batteries can thus replace the rotating inertia that was traditionally provided by big rotating power generation groups (e.g., coal plants). This inertia is essential to maintain a stable grid frequency. |
Energy Equity – Batteries as an enabler for further decentralization of power systems and enhanced public participation (energy democracy) For the customer EU average electricity price for household consumers lied at 21.3 ct€/kWh in 2020[2]. Battery prices have fallen by over 85% since 2010, enabling self-consumption technologies (PV + battery) to become cost-competitive. Now, these can eventually offer energy at a cheaper cost than that from the public grid (reaching the so-called grid parity). Self-consumption enables at the same time a high private and communal participation in the further development of the energy transition (thus contributing to the energy democracy). For the system/society When adding batteries to customer-sited PV installations, a smoother interaction with the grid is ensured, by decreasing both consumption peaks, as well as feed-in excess power spikes (peak shaving). This can reduce grid stress, and eventually avoid grid expansion. Hence, batteries can contribute to overall cost reduction, by optimizing grid operation, facilitating energy access for the most vulnerable social segments. Aside, increased competitivity of self-consumption and high self-sufficiency power supply technologies is especially relevant for those regions with high power generation or energy transport associated costs, like islands or remote rural regions. |
Environmental Sustainability – Batteries enable the integration of higher RES shares For the customer Adding a battery system to a private or public building for increased self-consumption can, in most of the European countries, depending on the energy mix, significantly reduce the weighted average of the emission factors of the consumed energy. The emissions associated with battery storage lie at about 50 g CO2eq per kWh of energy stored, while PV-generated energy has an associated footprint of about 30 g CO2eq/kWh. In comparison, energy from waste (biomass) sources has associated emissions of about 100 g CO2eq/kWh, and energy from gas firing or coal plants over 250 g CO2eq/kWh and 350 g CO2eq/kWh[3] respectively. For the system/society Energy storage systems provide flexibility to the electrical power system, as they can shift energy for a certain time window to match and balance supply and demand. Therefore, such technologies are an indispensable component for modern grids with high shares of variable renewable sources, to ensure matching the supply and demand power at all times. Batteries constitute one of the best alternatives for a large share of such needs (for energy shifts from milliseconds to days), while other technologies, e.g., power-to-gas, might be more competitive for seasonal storage of large energy volumes. |
As it can be seen, battery storage systems combined with customer-sited PV installations have a positive impact to each of the trilemma vertices.
What is the role of battery storage in the project REACT?
Innovative battery storage systems are a key element within the EU Horizon2020 research project REACT and are therefore installed in most of the involved demo sites throughout all pilot islands: La Graciosa, Spain; Inis Mór, Ireland; and San Pietro, Italy.
The main core innovation field investigated by the REACT consortium, in relation to battery storage technologies, relies on the integration of batteries within a central ICT platform (digitalization), that allows the installed battery storage systems to become a core element of the demand response capabilities of each of the REACT demo sites.
Thanks to the efforts put by the consortium partners, the required communication channels and interfaces will be implemented, which will enable extended monitoring and smart control capabilities to analyze and influence the performance of the batteries at each demo site.
The integration of several battery system products to the REACT platform is based on the well-known open-source energy management framework OpenMUC[4], or on existing product APIs (e.g., MIDAC API), and will provide the central ICT platform with beyond state-of-the-art control capabilities for the coordinated action of all energy storage systems.
For each of the customers, the integration with the platform allows for the use of the back-end services that the platform provides, including for example weather, energy generation, and consumption forecasts. With such forecasts, the battery control can be scheduled to optimize battery utilization. As an example, in Fig. 2, it can be observed that thanks to the usage of precise forecasting, the maximum export energy (as an example) can be decreased significantly.
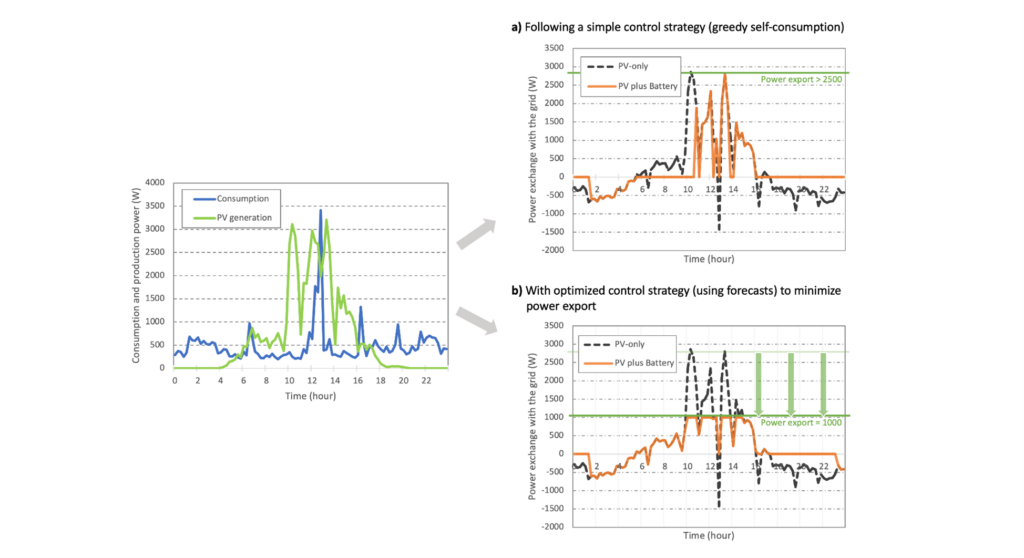
Fig. 2: Exemplary power balance plot for a June day at a household. On the left, the generation and consumption power profiles are shown. On the right, the resulting net export power balance (Ppv – Pload) is shown under two different control strategies for the battery. For this example, a 4.5 kWp of PV and a 4.5 kWh battery were assumed.
As a side effect, the aggregation of all these battery assets within a common platform allows for the energy service companies (ESCO) operating the ICT infrastructure to provide grid services to distribution and transmission system operators (e.g., frequency containment reserve), which can add up to the benefits of self-consumption and offer further revenue streams to end-customers.
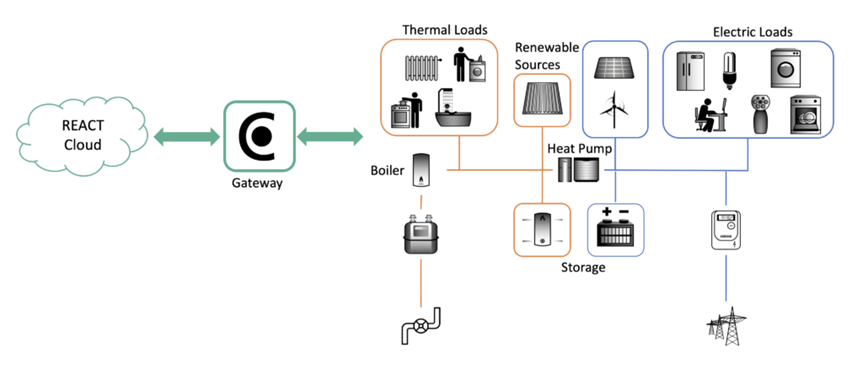
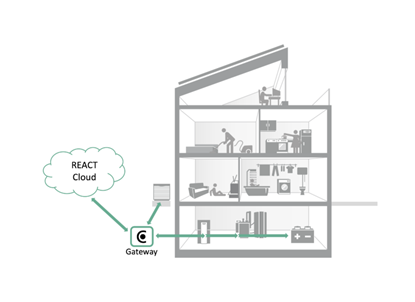
[MBL1]Copyright
[MBL2]This is an image generated by Fraunhofer ISE
Last but not least, REACT aims to compare the performance of several technologies, including lithium-ion, advanced lead-acid, and sodium-ion, along with other energy storage technologies (power-to-gas, for seasonal storage), to provide island communities with adequate technical insights about the best energy storage technologies that should be further deployed to enhance the energetic self-sufficiency of islands in an efficient and effective manner.
ANNEX: quick facts for the adoption of a battery system
If the presented arguments sounded convincing, and you would like to think about installing a battery system (and maybe joining the REACT platform as well?), we tried to collect a small guide of quick facts that should be taken into account at first.
What are the most common battery technologies? The most common technologies for residential storage of energy in Europe are lithium-ion (with more than 80% or recent installations), lead-acid, and redox flow batteries. Lithium-ion batteries keep improving significantly (including performance, safety, and cost-efficiency) and will probably be the dominant technology option for the next decade. Nonetheless, other novel technologies with salt-water-based electrolytes (more environment-friendly and earth-abundant materials) are already available and can become a competitor in terms of performance and costs in the near future. |
What are the main components? A battery storage system is mainly conformed by a battery pack (primarily containing the rechargeable electrochemical cells) and a power converter, including a battery charger and inverter, that allows the transformation of direct current (DC) to alternate current (AC). Other minor components include fuses and energy meters, along with other optional components. |
How is the equipment connected? Battery systems are usually connected to the main electrical switchboard from a building, like PV systems, and must be carried out by qualified technicians. Regarding the system topology (the connection between PV and battery), the main options are (i) DC-coupled, or (ii) AC-coupled. DC-coupled topologies are recommended in the case of simultaneous installation of PV and battery, while AC-coupled is preferred for battery systems installations that retrofit or are added to already existing PV systems. ![]() |
What is the right size of the battery? Although professional assessment is highly recommended for each use case, as the right sizing of PV battery systems heavily depends on regional and national conditions and or particular technical aspects from each building, a sizing estimation for enhanced self-consumption can be: First, the size of the PV (kWp) can be selected to reach an electricity yield (production) similar to the energy consumption per year, thus reaching a zero-energy balance with such PV capacity, battery storage with a similar capacity (kWh) than the selected PV size (approximately 1 kWh per kWp) is a good rule-of-thumb approximation for a correct sizing |
What is the volume of the battery system? Residential battery systems have normally a volume that is not greater than that of a dishwasher (or smaller), and can be mounted on the ground (normally, indoors) or hung on the wall. |
How much will it cost? As of 2020, and depending on the technology and size, a turnkey cost of about 1000 €/kWh can be reached for a typical residential storage system with a capacity between 5 to 10 kWh. |
[1] https://www.worldenergy.org/assets/downloads/World_Energy_Trilemma_Index_2020_-_REPORT.pdf
[3] Associated emission factors according to the latest values published by the Covenant of Mayors for Climate and Energy initiative (CoM). https://ec.europa.eu/jrc/en/publication/covenant-mayors-climate-and-energy-default-emission-factors-local-emission-inventories-version-2017
[4] www.openmuc.org
Lluis Millet Biosca (FHG ISE)